Black holes & Co. / Elementary tour part 1: Neutron stars and pulsars
Simultaneously, the star’s core region collapses. Within seconds, the core’s density increases to a point where the star’s plasma can no longer resist the pressure. The electrons and protons unite to form electrically neutral neutrons, and the core region evolves into an unbelievably dense ball of nuclear matter, no more than 20 kilometres (13 miles) in diameter, but with a mass greater than that of our sun: A neutron star is born. A pinhead’s worth of neutron star matter has more than twice the mass of the Queen Mary II, the world’s largest cruise ship.
A figure skater’s rotation speed increases dramatically when the arms and legs are pulled close to the body axis for a pirouette. By the same laws of physics, the contraction of a slowly rotating stellar core can lead to a neutron star that rotates a few hundred times every second. The interplay of rotation, magnetic field and a cloud of elementary particles which surrounds the neutron star can lead to an exciting consequence: The star can act like a cosmic light-house, with two narrow beams of radio waves pointing in opposite directions, sweeping in a circle with each rotation of the star. If the star is oriented in a way that one of those beams sweeps across the earth, then (as with a light-house) terrestrial astronomers will see a regular blinking, as sketched in the following animation:
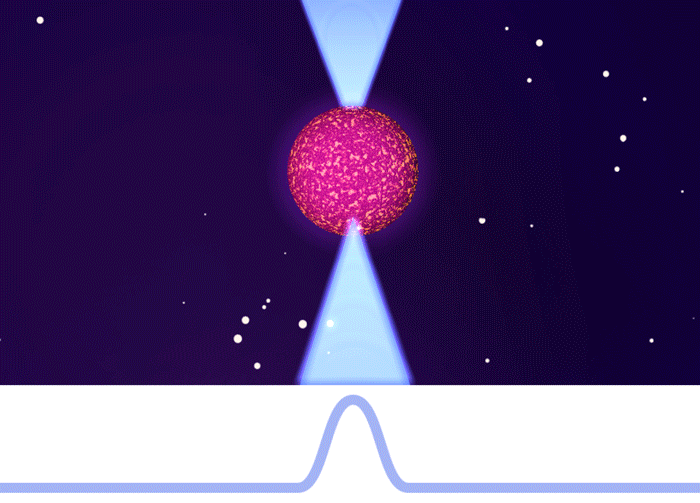
To radio astronomers, this type of neutron star appears as a pulsar, an object from which they receive a highly regular chain of radio pulses.
Due to their compactness, neutron stars are ideal cosmic laboratories for testing general relativity (especially when, as pulsars, they send out highly regular signals for astronomers to analyze): Close to a compact body, gravity is very strong, and under such conditions, the interesting effects of general relativity are very large. For instance, binary systems involving a neutron star can be used to test the influence of gravity on light (i.e., the radio light of the pulses) to high precision. Also, from such systems we have the first indirect evidence for the existence of gravitational waves (cf. PSR1913+16).