Third-generation gravitational-wave detectors
Starting in the 2030s, third-generation gravitational-wave observatories will eavesdrop on space. They are many times more sensitive than current detectors and could thus provide a range of new insights into the invisible side of the universe.
An article by Benjamin Knispel
The era of gravitational wave astronomy has begun – with Advanced LIGO and Advanced Virgo having observed 90 signals as of June 2022. In the coming years, these kilometer-sized and high-precision observatories, together with the Japanese KAGRA and the planned LIGO India, will detect many more gravitational-wave signals and provide new insights into the invisible side of the universe. Regardless, for some time now, scientists have been preparing the next generation of gravitational wave detectors – even larger and many times more sensitive observatories that will eavesdrop on the cosmos from the 2030s onwards.
While the space-based gravitational wave detector LISA is designed to pick up low-frequency space-time ripples that cannot be detected at the Earth’s surface, the so-called “third-generation” ground-based detectors will cover a frequency range similar to that of current instruments – with a sensitivity that is up to 10 times higher than Advanced LIGO and Advanced Virgo in their final stage of development. Two international projects are currently in preparation: in Europe under the name “Einstein Telescope” and in the U.S. as “Cosmic Explorer”. Both instruments will be much larger than the current facilities. However, their design differs significantly in some respects.
The Einstein Telescope
Like the Japanese KAGRA detector, the Einstein Telescope (ET) will be built underground. In suitable underground conditions, the influence of seismic vibrations that are always present on Earth is reduced. Just like an object is protected from external shaking and vibrations in a well-padded package, an underground detector would also be better isolated from man-made ground motion at the Earth’s surface if suitably “padded”. In addition, the so-called Newtonian noise is reduced by going underground. It originates from local low-frequency fluctuations of the terrestrial gravitational field (for example, due to atmospheric processes, seismic and acoustic waves, but also man-made changes), which move the end mirrors of the detectors and thus appear as an additional source of noise in the instrument. Currently, the suitability of three possible sites is being evaluated: The Meuse-Rhine Euroregion in the Belgium-Germany-Netherlands border triangle, the Mediterranean island of Sardinia, and the German part of Lusatia.
The ET’s tunnel system will have the shape of an equilateral triangle with 10-kilometer edges. Two adjacent tunnels will each contain a gravitational wave detector. The advantage of this arrangement is that each tunnel needs to be built only once, but can be used by two instruments. By operating three identical gravitational wave detectors in one location, additional information can be obtained from the observed signals that would otherwise only be available with multiple instruments that are far apart. Local disturbances can also be more easily identified as such, which increases the detection reliability.
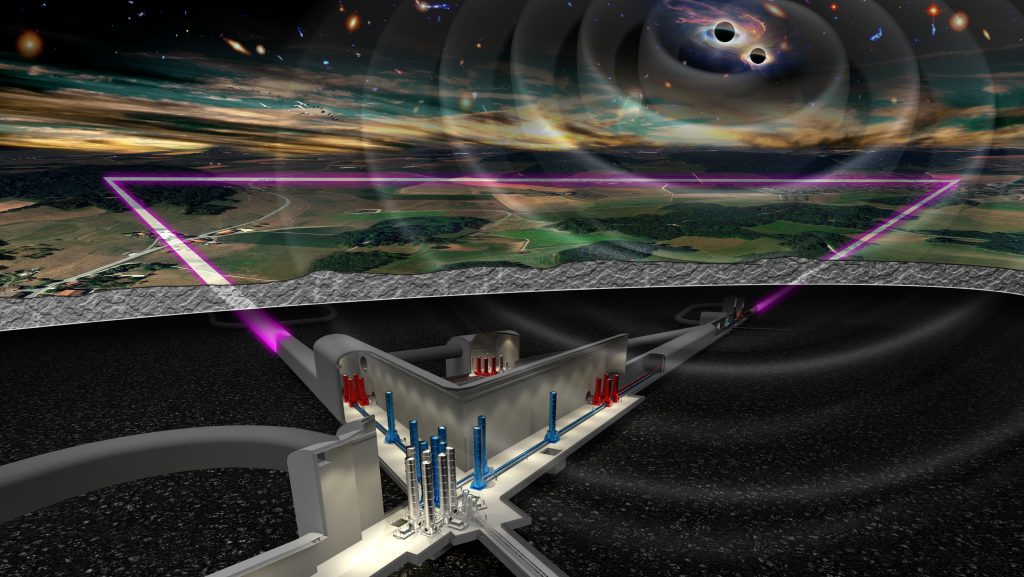
In addition, the ET will use two interferometers at each corner of the tunnel system to broaden the detectable frequency band, because technically, it is not possible to optimize one instrument for both frequency ranges. Thus, in case of the ET, one instrument (with low laser power and cryogenic mirrors) specializes in low-frequency gravitational waves (about 2 Hz to 40 Hz), and the other (with high laser power at room temperature) in those at higher frequencies (40 Hz to several kHz). In this “xylophone” configuration, the measurement data from both instruments are merged afterwards, so that the ET listens to space in a particularly broad frequency band and hears more of the gravitational-wave universe.
Cosmic Explorer
Cosmic Explorer is currently being planned essentially as a 10-times larger version of the LIGO detectors. The two arms of the detector are to be 40 kilometers long, and, as with LIGO, at right angles to each other. In the current development concept, a second site with arms that are 20 kilometers long is also planned. As with LIGO, Virgo and LIGO India, the arms will be located on the Earth’s surface – protected by covers from environmental influences. The advantage of such a design is that it allows the researchers to build on their previous experience and that it eliminates the technical expense of tunnel construction. Nevertheless, the above-ground construction of such a large detector is also challenging because the curvature of the Earth is clearly noticeable at these arm lengths. The above-ground construction also does not allow the sensitivity at low frequencies to be increased as much as with the ET.
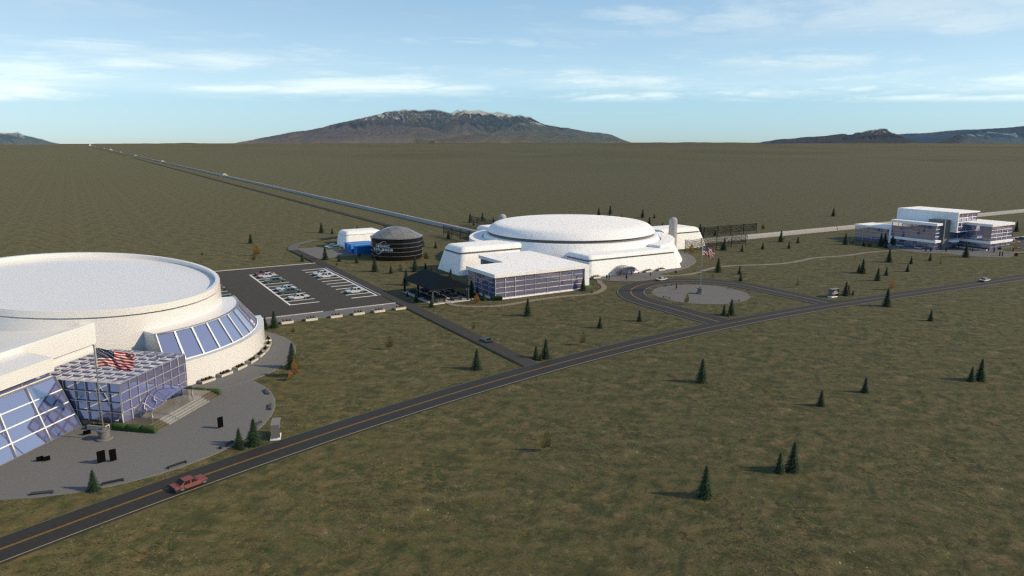
Listening more closely and deeper into space
Both third-generation detectors have an overall increased sensitivity to gravitational waves due to the longer arm length and the use of new technology. The spacetime waves stretch and compress space, thus, the more space in the arms, the more noticeable are the gravitational waves. At the same time, however, technical and fundamental physical noise superimposed on the gravitational-wave signals does not increase. As a result, more details become visible in the larger instruments, and weaker waves that previously remained completely hidden will appear for the first time. As a result, the rate at which gravitational waves are observed will be growing. While today a signal is heard every few days, the third generation of detectors will be listening to the gravitational-wave sound of the universe almost continuously.
The finer details of the waveform will reveal more about the source from which the wave was emitted. This will provide new information about the behavior of extremely dense matter in neutron stars and about the distribution of merger events on cosmic time scales, and it will facilitate new cosmological studies.
Certain signals will be detectable from nearly the entire universe with the next generation of detectors. The ET will detect mergers of stellar black holes whose gravitational waves were emitted some two hundred million years after the Big Bang. Cosmic Explorer, with slightly different frequency-dependent sensitivity, will hear signals from merging binary neutron stars from a similarly distant past.
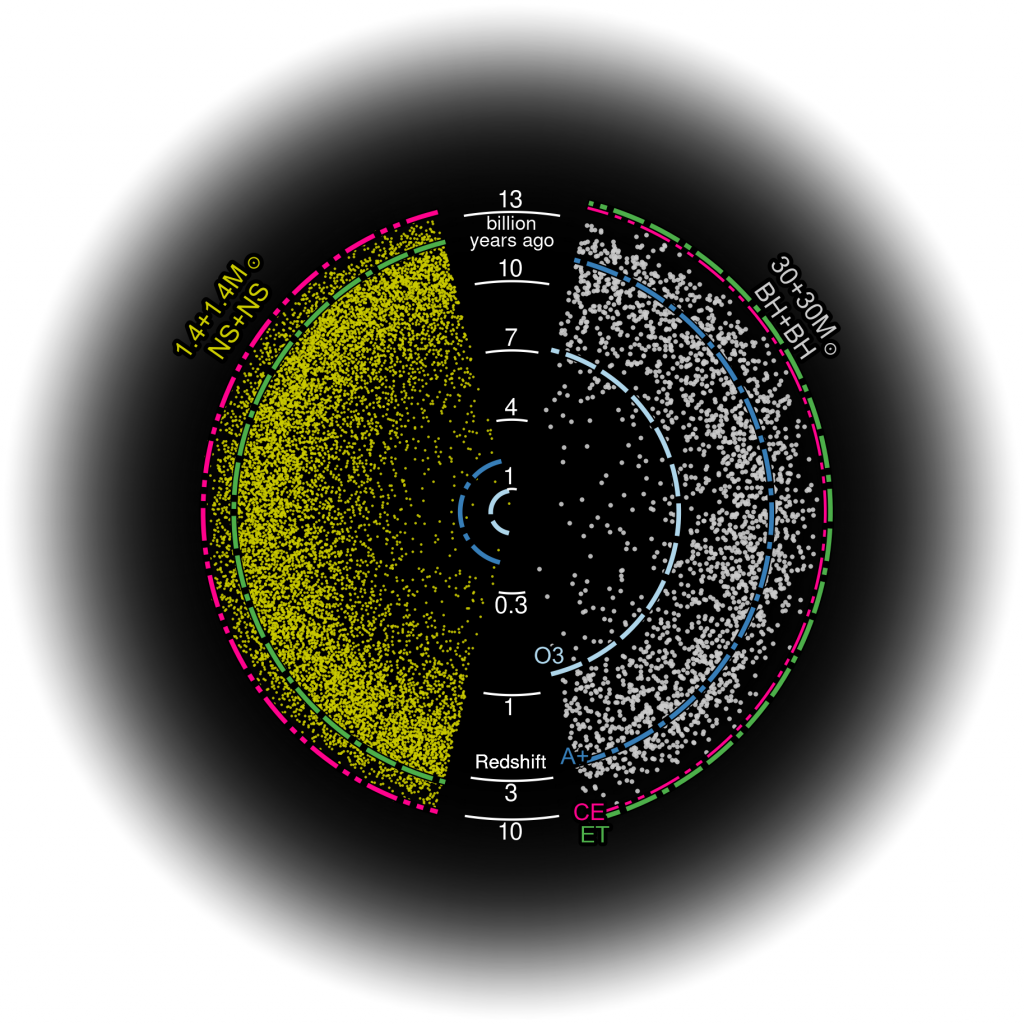
Because of their increased sensitivity at low frequencies, third-generation detectors will be able to detect the merger signals of intermediate-mass black holes (those with 100 to 10,000 solar masses). From these observations, researchers hope to gain new insights into how the even more supermassive black holes formed at the centers of galaxies.
Engineering Challenges
However, to enable these observations with significantly increased sensitivity, technical improvements beyond the mere increase of size are required. Increased arm length is the direct route to higher sensitivity, but it also requires larger vacuum systems and increased construction effort, especially if the facility is to be built underground. In addition, because the beam diameter of the lasers increases with longer travel distance, larger mirrors with smoother and more uniform mirror coatings are needed.
To minimize thermal noise, the end mirrors will be cooled to temperatures near absolute zero. But this comes with significant challenges. The heat deposited on the mirrors by the laser must be dissipated without causing additional noise. At these low temperatures, the special glass that has been used so far loses the good mechanical properties for which it was selected. New materials such as crystalline silicon or sapphire are being discussed as possible solutions, but they must be produced in sufficient size (the mirrors could weigh around 300 kilograms) and quality. This is a field of ongoing research. The production of appropriate highly reflective mirror coatings is also a major challenge.
The lasers for the third generation of detectors will also differ from those of current instruments. In addition to even higher powers of many hundreds of Watts, the new mirror and beam splitter materials will require switching to higher laser wavelengths. Scientists are currently developing and researching the required laser systems.
A global network
Even with the third generation, only an international network of detectors with comparable sensitivities can determine with high precision the celestial direction from which the gravitational waves are coming. And only then could other astronomical facilities reliably make follow-up observations. A third detector, in addition to the ET and Cosmic Explorer, in Earth’s southern hemisphere could complete such a network. Initial studies are already underway in Australia on the possible implementation of such an endeavor.
Astronomy with third-generation gravitational-wave detectors has the potential to investigate many unanswered questions in more detail, or even to answer them for the first time. Research is currently underway to solve the technical-scientific challenges posed by this new generation of detectors. Within just a few years, it will be decided where the next steps in gravitational-wave astronomy will lead.
Further Information
Colophon
is an astrophysicist and press officer at the Max Planck Institute for Gravitational Physics (Albert Einstein Institute) in Hanover, Germany.
Citation
Cite this article as:
Benjamin Knispel, “Third-generation gravitational-wave detectors” in: Einstein Online Band 14 (2022), 1002