Observation of Gravitational Waves from a Binary Black Hole Merger
Albert Einstein predicted their existence back in 1916, and on 14 September 2015 they were directly detected for the first time: Gravitational waves. Two large interferometric detectors of the LIGO Scientific Collaboration with major contributions from German researchers detected the signal known as “GW150914”. The waves originate from the merger of two black holes and are the first direct observation of these exotic objects.
An article by Peter Aufmuth
One hundred years ago, Albert Einstein predicted in his general theory of relativity the existence of gravitational waves. These ripples in spacetime propagate at the speed of light and are generated by changes of the mass quadrupole moment of the source over time. Gravitational waves cause very small relative changes in length, so Einstein thought it impossible to detect them. On September 14, 2015, gravitational waves have been observed directly for the very first time.
All modern instruments to measure the length changes caused by gravitational waves are laser interferometers. In these, laser light travels along two orthogonal paths after being split with a semi-transparent mirror. At the end of those paths (the “arms” of the interferometer) are mirrors that send the light back. At an output photodetector both laser beams are overlapped. Depending on the length difference between the two arms, the light waves are “in sync” and are amplified (“constructive interference”) or they cancel out, in the extreme case totally (“destructive interference”).
The longer the arms of the interferometer, the better the effects of a gravitational wave can be measured. The length changes are a fraction of the arm length l, h = dl/l, where dl is the change of the arm length caused by the gravitational wave. For typical astrophysical sources the relative arm length change h has a value of 10-21 or smaller.
Today, there are four large interferometers in the world for the detection of gravitational waves. Two are in the USA, each with four kilometre long arms , in Hanford, Washington, and in Livingston, Louisiana. They are seperated by 3000 kilometres. In Europe, there is the French-Italian Virgo project with an arm length of 3 kilometres and GEO600, a German-British project with an arm length of 600 metres. From 2002 to 2010 first common observation runs took place, but up to now the instruments’ sensitivities were too low for the direct observation of gravitational waves. All these projects work together within the LIGO Scientific Corporation (LSC) and in the Virgo Collaboration. These include more than one thousand scientists at about one hundred institutions.
Since late 2015 there is an improved version of the LIGO detectors: => aLIGO (= advanced LIGO). Between 2010 and 2015 technological improvements developed at GEO600 have been installed at the LIGO detectors, e.g., a laser with higher power. This laser has been developed by the Albert Einstein Institute Hannover and the Laser Zentrum Hannover. It produces an output power of 180 W and an amplitude steady to a billionth. Additionally, the aLIGO mirrors have been suspended at glass fibres with a procedure developed at the Institute for Gravitational Research of the University of Glasgow. This means that mirror and suspension are of the same material (“monolithic suspension”) leading to a much better reduction of thermal disturbances. Furthermore with another mirror the output signal can be superimposed with itself (“signal recycling”). This technique has been tested first at GEO600 and has been shown to enable a further improvement of the detector sensitivity.
Found in the first observation run
With all these improvements the LIGO detectors were expected to start the first observation run (O1) on September 18, 2015, but in fact started a little earlier on September 10, 2015. At September 14, a signal was observed (GW150914) at both aLIGO detectors. Scientists of the Max Planck Institute in Hannover were the first to see it becauseit was still night in the USA.
![Visualization of the merging black holes, their distance and velocity and the wave form from numerical solutions of the theory of relativity. / [Image: LIGO / Redesign: Daniela Leitner] Visualization of the merging black holes, their distance and velocity and the wave form from numerical solutions of the theory of relativity.](https://www.einstein-online.info/wp-content/uploads/gravitational_waves_Binary_Black_Hole_Merger_Ligo_©_Ligo_Redesign_Daniela_Leitner_for_Einstein-Online.jpg)
On top: Estimated gravitational wave amplitude of GW150914 at the Hanford detector. Above that are the Schwarzschild horizons of both merging black holes shown as calculated numerically from the general theory of relativity. Below: The effective distance of the black holes in units of Schwarzschild radii RS and the relative velocity in units of the speed of light. [Image: LIGO / Redesign: Daniela Leitner]
Exact waveform models for reference and discovery
In order to uncover the gravitational waves in the data, scientists at the AEI are calculating a whole series of possible forms of gravitational waves which arise when black holes or neutron stars merge. The resulting models are then compared with the observed data.
Only in this way was it possible to declare a true discovery in the GW150914 data with a statistical significance of 5 sigma – and even to make reliable statements about the nature of the merged objects.
Thorough analysis of the signal
GW150914 was observed by both aLIGO detectors in Hanford and in Livingston. It lasted less than half a second and increased during this period in frequency and amplitude. The maximum amplitude of the relative change in length was about 10-21. An exact analysis of the signal based on the theory of general relativity showed that it came from two merging => black holes with 29 and 36 solar masses, which merged 1.3 billion light years from Earth. After merger the resulting black hole had a mass of 62 Suns. During the merger three solar masses had been emitted in gravitational waves. Most of the energy had been released in the last 0.2 seconds – gravitational waves were emitted with a power ten times larger than the luminosity of all stars in the observable universe.
How certain is it that GW150914 really was a gravitational wave? Firstly, both aLIGO detectors observed the signal with a time delay of 7 ms – the time of flight for gravitational waves between both detectors is at most 10 milliseconds. Secondly, both signals show the same wave form, which is to be expected for an astrophysical source in view of nearly the same alignment of the detectors.
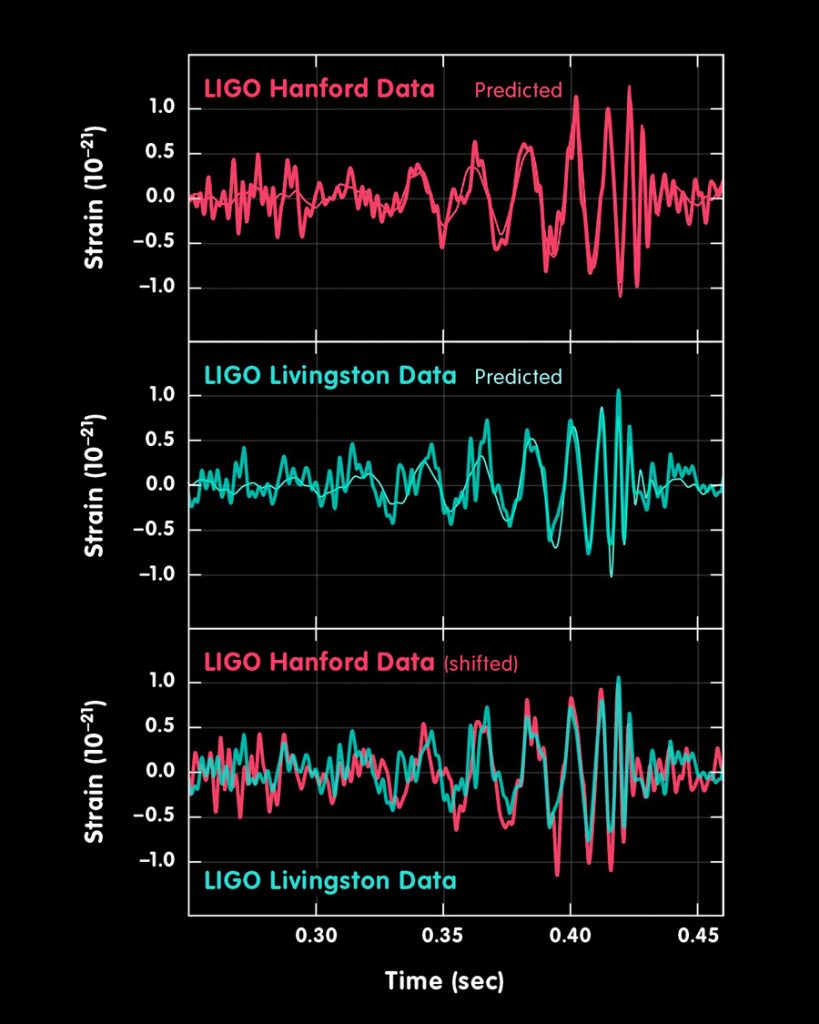
The gravitational wave signal of GW150914 as seen in Hanford (H1) and Livingston (L1), over time (measured in seconds). GW150914 arrived first at H1 and then 7 milliseconds later at L1 and is compared with numerical solutions. The lowest row shows the H1 data shifted by 7 milliseconds and inverted because of the relative orientation of both detectors. [Image: LIGO / Redesign: Daniela Leitner]
Nevertheless, it is possible that a signal with the correct shape and time delay occurs by pure chance out of the random noise of the two detectors. In order to determine the propability that such a strong signal occurs accidentally one has to take sufficiently long observations of the random detector noise and analyze it carefully. This analysis of the measurement period containing GW150914 resulted in the statement that such a strong signal could be produced by accidental fluctuations only once in 200,000 years. Such an error rate can be expressed as statistical significance as in particle physics. For GW150914 this significance is more than five standard deviations and the signal thus meets the strong demands for a real astrophysical event.
Currently the detectors are three times more sensitive than before – at lower frequencies even more. Within the next two years this sensitivity is expected to be enhanced by a factor of ten over the entire frequency band. Then one should see further sources, like the merger of two neutron stars.
Further Information
This first observation of gravitational waves was awarded the Nobel Prize in Physics in 2017.
The first two observation runs of LIGO and Virgo resulted in the catalogue GWTC-1 published in 2019.
Reference
- Observation of Gravitational Waves from a Binary Black Hole Merger
B. P. Abbott et al. (LIGO Scientific Collaboration and Virgo Collaboration)
Phys. Rev. Lett. 116, 061102
Colophon
is a scientist at the Albert Einstein Institute in Hannover who is involved in the maintenance and development of the gravitational wave detector GEO600.
Citation
Cite this article as:
Peter Aufmuth, “Observation of Gravitational Waves from a Binary Black Hole Merger” in: Einstein Online Band 10 (2016), 10-1001