Observing gravitational waves in space with LISA
In 2035, the Laser Interferometer Space Antenna (LISA) is scheduled to be launched into space as a mission of the European Space Agency (ESA). It will observe previously inaccessible gravitational waves from a large range of new sources.
An article by Benjamin Knispel
Earth-based instruments have already observed many dozens of gravitational-wave signals from merging compact objects such as binary systems of black holes and neutron stars. Their future upgrades and third-generation successors will detect many thousands more such signals from almost the entire universe and search intensively for signals from other sources (such as isolated rapidly rotating neutron stars).
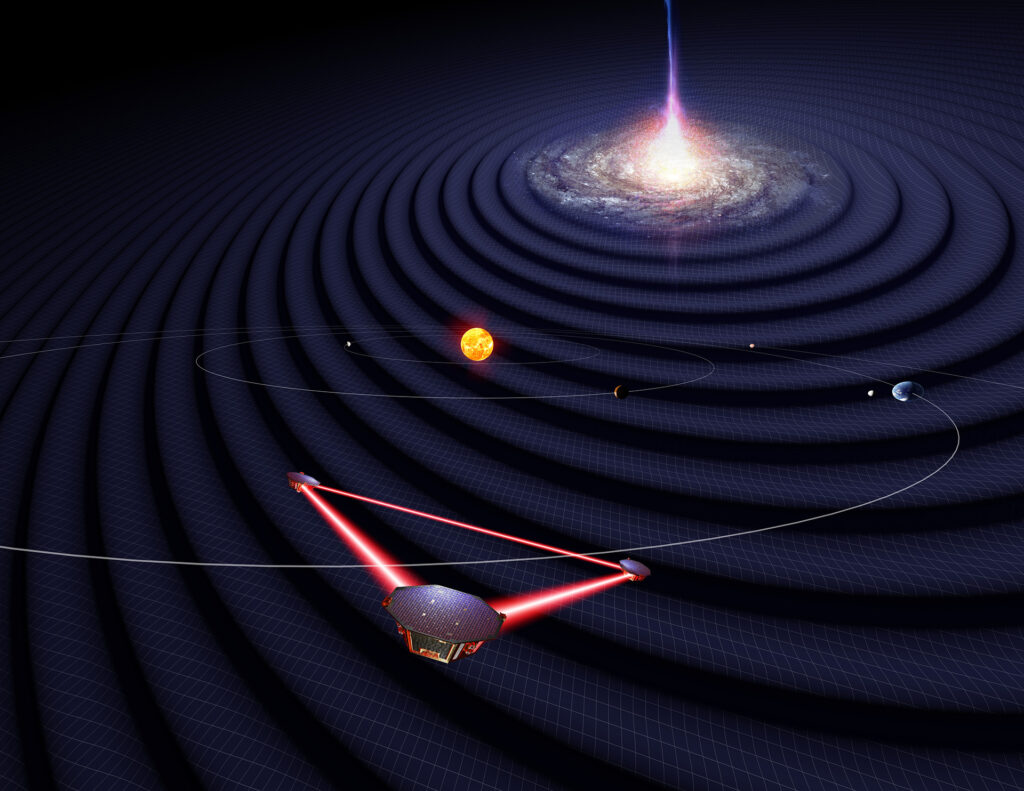
Why in space?
However, the Earth sets fundamental limits for the observation of gravitational waves at low frequencies. Firstly, detectors significantly larger than our home planet are required. Secondly, terrestrial seismic activity – constant natural vibrations – interferes with any measurements. At high frequencies, it can be technically suppressed so that it does not interfere with the observations. However, at low frequencies of less than one Hertz (less than one oscillation per second), this is not possible. Therefore, gravitational waves at lower frequencies cannot be observed on Earth.
A gravitational-wave detector in space does not have these difficulties: for one thing, there is no seismic activity in space. Secondly, there is ample room for very large detectors to observe low-frequency gravitational waves. The “Laser Interferometer Space Antenna” (LISA), a mission of the European Space Agency (ESA), will be such a space-borne instrument, consisting of three satellites. The satellites are currently being constructed and prepared for launch in 2035.
A laser triangle in space
LISA will be the largest astronomical observatory ever built. Its satellites will fly in a triangular formation, with 2.5 million kilometer long sides. That is more than six times the distance between the Earth and the Moon. The satellites will follow the Earth at a distance of around 50 million kilometers on their orbit around the Sun. The satellite triangle is inclined at an angle of 60° to Earth’s orbit.
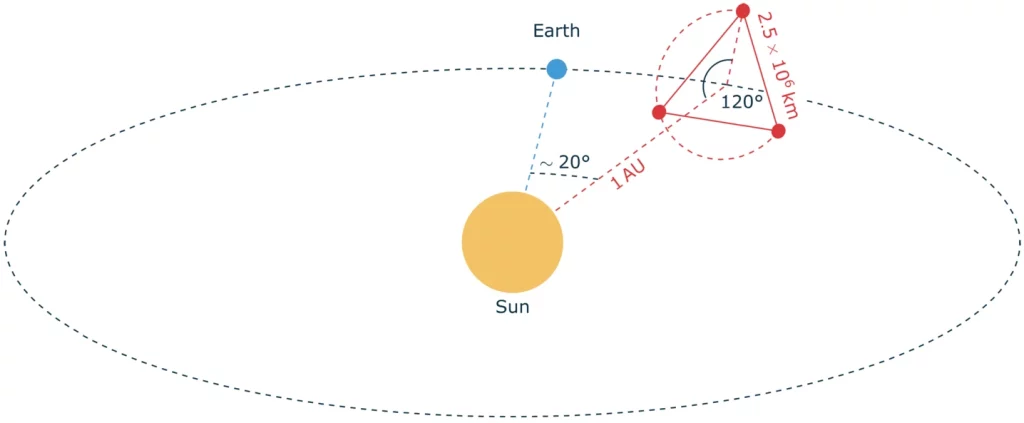
Image: CC-BY 4.0 Branchesi, M., Falanga, M., Harms, J. et al. Lunar Gravitational-Wave Detection. Space Sci Rev 219, 67 (2023).
Free-falling test masses on board each individual satellite move through space only under the influence of gravity – on a geodetic line. These test masses are 4.6 centimeter cubes made of a gold-platinum alloy that is hardly affected by magnetic fields. The satellites follow these test masses by precisely monitoring their position within the spacecraft using electric fields and laser interferometers. The satellites correct their position and speed relative to the test masses using sensitive thrusters.
The satellites shield external forces on the test masses (with the exception of the effects of gravity and gravitational waves which cannot be shielded). If, for example, solar wind or the radiation pressure of the sunlight push on the satellites, this is registered as a change in distance between the satellites and their test masses. The satellites compensate for this by actuating their thrusters, which generate a counterforce to the external disturbance. The result: the test mass inside the satellite continues to follow its geodetic line undisturbed.
Observing tiny length changes
Gravitational waves change the distance between the test masses in the three satellites. Typical gravitational waves in the frequency band observed by LISA are noticeable as motions of the test masses by a few tens to a few hundred trillionths of a meter (picometers).
In order to observe gravitational waves, LISA will therefore act as a giant interferometer to measure the distance between the test masses with picometer precision. As with the terrestrial detectors, which are based on the principle of a Michelson interferometer, LISA uses near-infrared laser light.
The arm length of the LISA constellation, which is around one million times longer than that of the terrestrial detectors, requires a change of the interferometric measuring principle. This is because over the 2.5 million kilometer distance, even an initially collimated laser beam expands to a diameter of several kilometers! At the distant satellite, it is received with a telescope aperture of 30 centimetres, so that only a tiny fraction (a few hundred picowatts) of the two watts of emitted laser light power is received. The direct superposition of laser beams that have passed through the measuring paths, as used in terrestrial detectors, is not possible with LISA.
Interferometry with a trick
For the laser-based detection of the changes in the distances between the free-falling test masses caused by gravitational waves, LISA instead relies on a transponder principle: LISA uses an interferometer on board each satellite to continuously measure the phase difference between the weak laser beam received from a distant satellite and the strong laser beam emitted by the local satellite. This measurement data is transmitted to Earth and, in addition to the desired gravitational-wave signals, contains additional interference: 1) a significantly stronger instrumental noise and 2) an additional phase shift resulting from the relative orbital motion of the satellites (because the satellite triangle cannot remain perfectly equilateral in the long term). When processing these data on Earth, these disturbances are removed, in order to identify the significantly weaker gravitational-wave signals.
LISA is a flexible measuring instrument: by cleverly combining the individual measurements over different distances between two satellites, different “virtual” interferometer configurations can be realized in order to optimize the sensitivity to gravitational waves. It is also possible to select combinations that have no sensitivity to gravitational waves. These can help to characterize the instrument itself.
Expanding the gravitational-wave spectrum
LISA will study much slower oscillations of space-time than terrestrial detectors, which observe gravitational waves in the range of a few Hertz to a few thousand Hertz. Just as radio telescopes, gamma-ray observatories, optical telescopes and X-ray satellites complement each other, LISA will open up a part of the gravitational-wave spectrum that was previously inaccessible to terrestrial instruments by observing gravitational waves with frequencies between 0.1 millihertz and 0.1 Hertz. The frequency range observed by LISA is also at significantly higher frequencies than the gravitational waves in the nanohertz range that pulsar timing arrays search for.
This observation of multiple frequency bands offers advantages: Cosmic sources that emit gravitational waves in a very wide frequency range can be observed more comprehensively and with independent observatories. Other sources that only emit gravitational waves in the millihertz range can now be detected for the first time with LISA. In this range, the universe harbors many sources of strong gravitational waves: Binary systems of supermassive black holes, small black holes orbiting and falling into more massive black holes, and close binary stars in our Milky Way. LISA will be able to observe binary systems of black holes with stellar masses several centuries before they merge. A gravitational-wave background and possibly completely new and unexpected sources are also on the list of potential future discoveries.
LISA as an early-warning system for terrestrial observatories
In addition, the observation of low-frequency gravitational waves from space opens up new possibilities for multi-messenger astronomy, i.e. the joint observation of astronomical objects with gravitational waves, electromagnetic waves and cosmic particles. This is because LISA can observe binary systems of black holes, whose merger signals are measured by terrestrial gravitational-wave detectors, up to several centuries earlier and thus alert the terrestrial observatories in advance in some cases. In addition, observations so long before their merger also provides new insights into the formation of the binary systems: While terrestrial observatories only “see” the period shortly before the merger, LISA will already be observing how the black holes approach each other, when they are still relatively far apart and their orbits show clear traces of their formation process and their surroundings. With LISA, the eccentricity of the orbits and the influences of dense gas disks or a nearby massive black hole can be determined more precisely than is possible with terrestrial detectors. These observations should allow insights into the formation mechanisms of these black holes.
In some cases, it should even be possible to observe the inspiral of the black holes with LISA and their merger with the future terrestrial detectors. The first signal observed by LIGO (GW150914) would have been detected with LISA more than 5 years before the merger! This kind of advance notice can enable subsequent targeted observation with third-generation terrestrial detectors. In addition, the precise determination of the expected merger time and sky position from LISA data will allow further observations with other astronomical observatories.
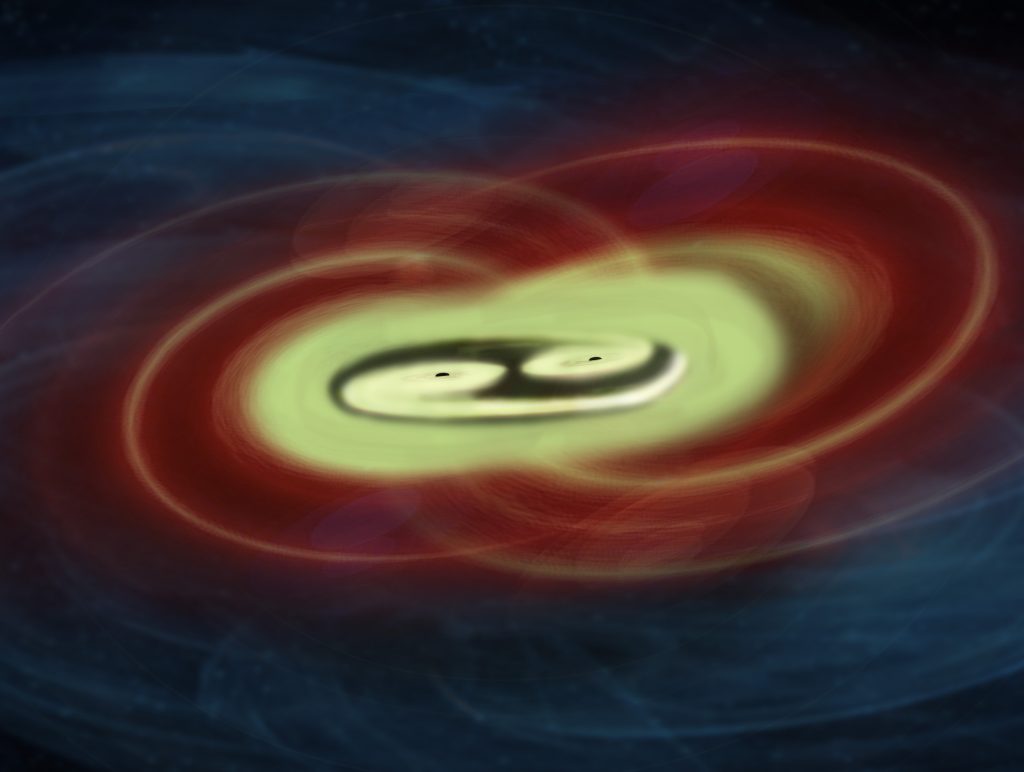
Gravity giant pairs
In addition to binary black hole systems with stellar masses whose mergers are observed by terrestrial detectors, there are true gravitational giants: supermassive black holes with masses millions of times greater than the ones observed so far. LISA will observe gravitational waves from mergers of such black holes from a large part of the observable universe. Binary black hole systems with total masses of 10,000 to 10 million times that of our Sun can be observed by LISA far back into the cosmic past to the beginning of galaxy formation.
Depending on the total mass of the binary, LISA observes the inspiral and merger over a period of several hours to several weeks. During this time, the observations can be used to determine the masses of the black holes and in which direction in the sky and at what distance from Earth they are located. The scientists can also obtain information about the angular momentum of the black holes.
Observing the gravitational waves of binary systems of massive black holes enables the study of the formation, growth and evolution of galaxies. This is because these binary systems are formed when galaxies merge, and they exist in their central regions. LISA will investigate when, how and from which objects the massive black holes were formed, how they grew, what role the galaxies that host them play and whether there is a connection to the lighter black holes that terrestrial gravitational-wave detectors already observe. The mission will shed light on previously unexplored areas of the universe and will provide the first census of this hitherto understudied population.
Unequal cosmic couples
Merging black holes often have similar masses. However, there are also systems where this is not the case. If the heavier of the merging objects is at least a factor of 1000 (and up to a factor of 1,000,000) more massive than the lighter one, things get complicated: The smaller object – which can be a lighter black hole, a neutron star, a brown dwarf or a normal star – orbits the heavier black hole for a long time on a complex, elliptical orbit with a changing inclination. The orbit is determined by many effects of general relativity.
LISA can make unique observations of these systems. Due to the large number of orbits observed, the properties of the unequal pair can be determined very precisely: What masses do both objects have? How fast does the heavy black hole rotate around its own axis? What is the inclination and eccentricity of the orbit – i.e. how strongly elliptical is it? How far away is the system from Earth? Researchers can use this data to estimate the number of such objects in the universe, and to investigate how they formed and evolved.
It is expected that these so-called “extreme mass ratio inspirals” (EMRIs) occur in the busy parts of the universe: the centers of galaxies or in dense star clusters. External influences on the merging binary system should leave their traces in the gravitational waves. This would provide a new opportunity to study the distribution of gas clouds, dark matter and stars in the vicinity of the heavy black hole.
Close binary stars in our home galaxy
Researchers assume that there are around 10 million close binary star systems in our Milky Way that, due to their orbital motion, emit gravitational waves in the frequency range that LISA will observe. Several dozen binary systems are already known in our galaxy whose gravitational waves LISA is guaranteed to observe. Because of their known orbital periods and distances from Earth, these verification objects are known to emit strong gravitational waves in LISA’s observation band. They will be used to test and calibrate the instruments and data analysis after the mission begins.
In most cases, the gravitational waves are too weak to identify a specific binary system as their origin. Just like identifying an individual voice amongst chatter is hard, the many binary systems generate a gravitational wave “murmur” that cannot be disentangled. The loudest signals from around 10,000 binary stars – most of them consisting of two white dwarfs – can be identified individually with LISA. A few hundred of these should also be observable in the electromagnetic spectrum.
LISA will measure gravitational waves from binary systems with very short orbital periods (<15 min) throughout the Milky Way and thus map their distribution. These observations will extend to areas that are difficult or impossible to reach with existing observatories. They will complete the picture of our galaxy and will allow researchers to indirectly determine the mass of all stars in the Milky Way.
The gravitational-wave background is buzzing
The gravitational-wave background generated by the binary systems in our galaxy is so “loud” that it can mask other gravitational-wave signals in a certain frequency range and exceed the instrumental noise. Binary systems and their mergers outside of our Milky Way are likely to produce a much weaker background. If LISA observes this signal, conclusions can be drawn about the frequency of these mergers in the course of cosmic history.
LISA could also observe gravitational waves from the very early universe as a background signal. Possible causes for this would be exotic phenomena beyond the standard model such as the inflationary phase, cosmic strings or primordial black holes. Here, too, LISA can make unique contributions to improving our understanding of these objects and verifying their hitherto only postulated existence.
LISA will enable precise tests of Einstein’s general theory of relativity and its extensions. Surprises may await and completely new signals may emerge from the analysis of LISA data that do not correspond to the expectations and physical models of the researchers – and leave them puzzled. The instruments and data analysis are therefore designed in such a way that it is possible to detect such “unmodeled” sources.
LISA on its way into space
ESA’s LISA Pathfinder mission between 2015 and 2017 demonstrated that LISA’s extremely demanding measurements will succeed. Key technologies such as laser interferometry, control of the test masses and the sensitive thrusters for LISA were tested, their functionality demonstrated and the results even exceeded LISA’s precision requirements.
LISA’s interferometric measurement principle has been used on board the two GRACE Follow-On satellites since summer 2018. The satellites orbit the Earth and use laser interferometry to measure changes in their mutual distance with nanometer precision. The observed distance changes contain information about the Earth’s gravitational field and how it changes over time, which in turn makes it possible to track the melting of polar ice masses and changes in global groundwater levels. LISA technology has therefore already found practical applications in climate research even before the launch of the actual mission.
At the beginning of 2024, ESA firmly anchored the LISA mission in its program and confirmed that it is mature and its development can continue as planned. This marked the beginning of the implementation phase for LISA, in which the selection of an industrial prime contractor and the preliminary and critical design review will be the next important milestones before the planned launch in 2035. The Max Planck Institute for Gravitational Physics (Albert Einstein Institute) at the Hanover and Potsdam sites plays a leading role in the implementation of the mission: in the development of key hardware components as well as in the modeling of the sources, data analysis and evaluation of the scientific results.
Further Information
An Einstein Online article about LISA from the year 2010 by Peter Aufmuth can be found here.
Colophon
is an astrophysicist and press officer at the Max Planck Institute for Gravitational Physics (Albert Einstein Institute) in Hanover, Germany.
Citation
Cite this article as:
Benjamin Knispel, “Observing gravitational waves in space with LISA” in: Einstein Online Band 15 (2024), 1001